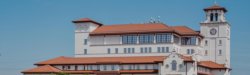
View Profile Page
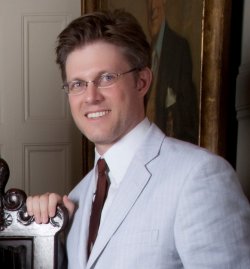
David Talaga
Associate Professor, Chemistry and Biochemistry, College of Science and Mathematics
- Office:
- Richardson Hall 225L
- Email:
- talagad@montclair.edu
- Phone:
- 973-655-7167
- vCard:
- Download vCard
Specialization
Amyloid (aggregated form of protein that accumulates in Alzheimer’s Disease, Parkinson’s Disease, Type II Diabetes, Spongiform Encephalopathies and others) formation mechanisms
Approaches include: Single molecule fluorescence lifetime, Solid state nanopores, Electrochemical impedance spectroscopy, Interfacial effects on proteins, Novel methods of global data analysis
Office Hours
Fall
- Monday
- 9:30 am - 10:30 am
- Thursday
- 10:00 am - 11:00 am
- 1:30 pm - 2:30 pm
Spring
- Monday
- 2:30 pm - 3:30 pm
- Thursday
- 11:30 am - 12:30 pm
- 3:00 pm - 4:00 pm
Summer
- Monday
- 9:00 am - 4:30 pm
- By appointment
- Tuesday
- 9:00 am - 4:30 pm
- By appointment
- Wednesday
- 9:00 am - 4:30 pm
- By appointment
- Thursday
- 9:00 am - 4:30 pm
- By appointment
- Friday
- 9:00 am - 4:30 pm
- By appointment
Links
Montclair State does not endorse the views or opinions expressed in a faculty member's webpage or website. Consistent with the principles of academic freedom, the content provided is that of the author and does not express the opinions or views of Montclair State University.
Research Projects
Protein folding and aggregation
The study of amyloid structure and growth has been motivated by their implication in many human diseases.
There are more than 20 diseases associated with excessive deposits of amyloid plaques in the affected tissue or organ including Alzheimer's disease (AD), Parkinson's disease (PD), type II diabetes, and spongiform encephalopathies. In these disease states, proteins that are normally soluble undergo aggregation to form various intermediates and amyloidogenic species. These species subsequently assemble to generate insoluble fibrils that accumulate in the affected tissues or organs. A detailed understanding of amyloid growth mechanisms will allow new approaches to the prevention of amyloid formation and better diagnostics for early detection of amyloidogenic diseases. A molecular-level mechanism of how the different amyloid species interconvert is the goal of this project. There are many species of amyloid particles present physiologically. Our single molecule studies aim to classify the species involved in amyloid formation according to size, shape, kinetic reactivity, and monomer 2° and 3° structural information. A molecular-level mechanism of amyloid growth must include details as to when the protein misfold occurs and how it is influenced by the dynamics of protein structure. To determine the physical interactions and structural changes involved in the amyloid assembly mechanism, we study effect of environmental variables such as temperature, pH, helix promoting solvents, denaturants, and reducing agents. The environmental effect on aggregation is expected to be species-dependent reflecting a possible hierarchy of structural interactions.
Oligomer Stability and Fibrilization in Polypeptide Drugs
This research aims to elucidate the role of oligomer formation in the stability of polypeptide drugs, focusing on factors influencing the propensity of these drugs to form amyloid fibrils. Key questions include understanding how resistant or prone different oligomers are to fibrilization and how polypeptide drugs can be formulated to avoid this process. By identifying the conditions and characteristics that lead to fibril formation, the study seeks to enhance the stability of polypeptide drugs, making them safer and more effective for therapeutic use.
Polypeptide drugs have gained significant importance in recent years due to their ability to modify the activity of endogenous hormone receptor. However, their tendency to form amyloid fibrils poses a major challenge, impacting both their efficacy and safety. Understanding the mechanisms underlying oligomer stability and fibrilization is crucial for developing stable formulations.
Our studies employ a combination of biophysical techniques to investigate oligomer stability and fibrilization in polypeptide drugs. Size exclusion chromatography is used to analyze oligomer distributions, while Thioflavin T fluorescence assays detect amyloid fibril formation. Circular dichroism and intrinsic polypeptide fluorescence provide information on structural changes and stability. Advanced techniques such as single molecule fluorescence and interfacial spectroscopy offer insights into molecular interactions and surface properties. Dynamic light scattering is utilized to measure particle sizes and aggregation states, providing a comprehensive understanding of the factors influencing fibrilization.
Preliminary results indicate that preformed seeds resulting from fibrillogenic stress are a key antecedent for fibrilization of polypeptide drugs. This finding suggests that targeting the formation and stability of these seeds could be a potential strategy for preventing fibrilization and improving drug stability.
The findings from this research have broad implications for the development of polypeptide drugs. By understanding the mechanisms of fibril formation, pharmaceutical companies can design more stable drug formulations, potentially reducing the risk of fibril-associated immunogenic side effects and improving therapeutic outcomes.
Identification of proteins by solid state nanopore measurements
Our preliminary studies on protein translocation in silicon nitride nanopores have shown that the nanopore translocation signals are sensitive enough to distinguish the folding state of a protein and resolve different proteins. We now seek to develop nanopore technology to distinguish and count protein molecules in their unfolded state based on their nanopore translocation electrical current signal. How the nanopore protein translocation electrical signal depends on the primary sequence, pH, and voltage will be studied for the selected SNase mutants.
The nanopores we use are fabricated from silicon nitride and are 5–30 nm in length and ∼4 nm in diameter. A protein molecule transiting a nanopore transiently block part of the current. The blockage is proportional to the local excluded volume Λ(t) of the protein molecule. The translocation time depends on the electrical potential barrier(s) to exiting the pore that arise due to distribution of primary charges on the peptide chain. Thus both primary observables in nanopore measurements are sequence-dependent. We seek to exploit this protein sequence dependence of the nano pore signals to distinguish proteins in solution to enable new experimental approaches to protein expression profiling applicable at the single cell protein level.
A preliminary analysis of all the protein sequences in the human genome suggests that coupled with current generation capillary electrophoresis and microfluidic-based isoelectric focussing, nanopore analysis could discriminate most of the proteins if key challenges can be met.Our preliminary studies on protein translocation in silicon nitride nanopores have shown that the nanopore translocation signals are sensitive enough to distinguish the folding state of a protein and resolve different proteins. We now seek to develop nanopore technology to distinguish and count protein molecules in their unfolded state based on their nanopore translocation electrical current signal. How the nanopore protein translocation electrical signal depends on the primary sequence, pH, and voltage will be studied for the selected SNase mutants. The nanopores we use are fabricated from silicon nitride and are 5–30 nm in length and ∼4 nm in diameter. A protein molecule transiting a nanopore transiently block part of the current. The blockage is proportional to the local excluded volume Λ(t) of the protein molecule. The translocation time depends on the electrical potential barrier(s) to exiting the pore that arise due to distribution of primary charges on the peptide chain. Thus both primary observables in nanopore measurements are sequence-dependent. We seek to exploit this protein sequence dependence of the nano pore signals to distinguish proteins in solution to enable new experimental approaches to protein expression profiling applicable at the single cell protein level. A preliminary analysis of all the protein sequences in the human genome suggests that coupled with current generation capillary electrophoresis and microfluidic-based isoelectric focussing, nanopore analysis could discriminate most of the proteins if key challenges can be met.